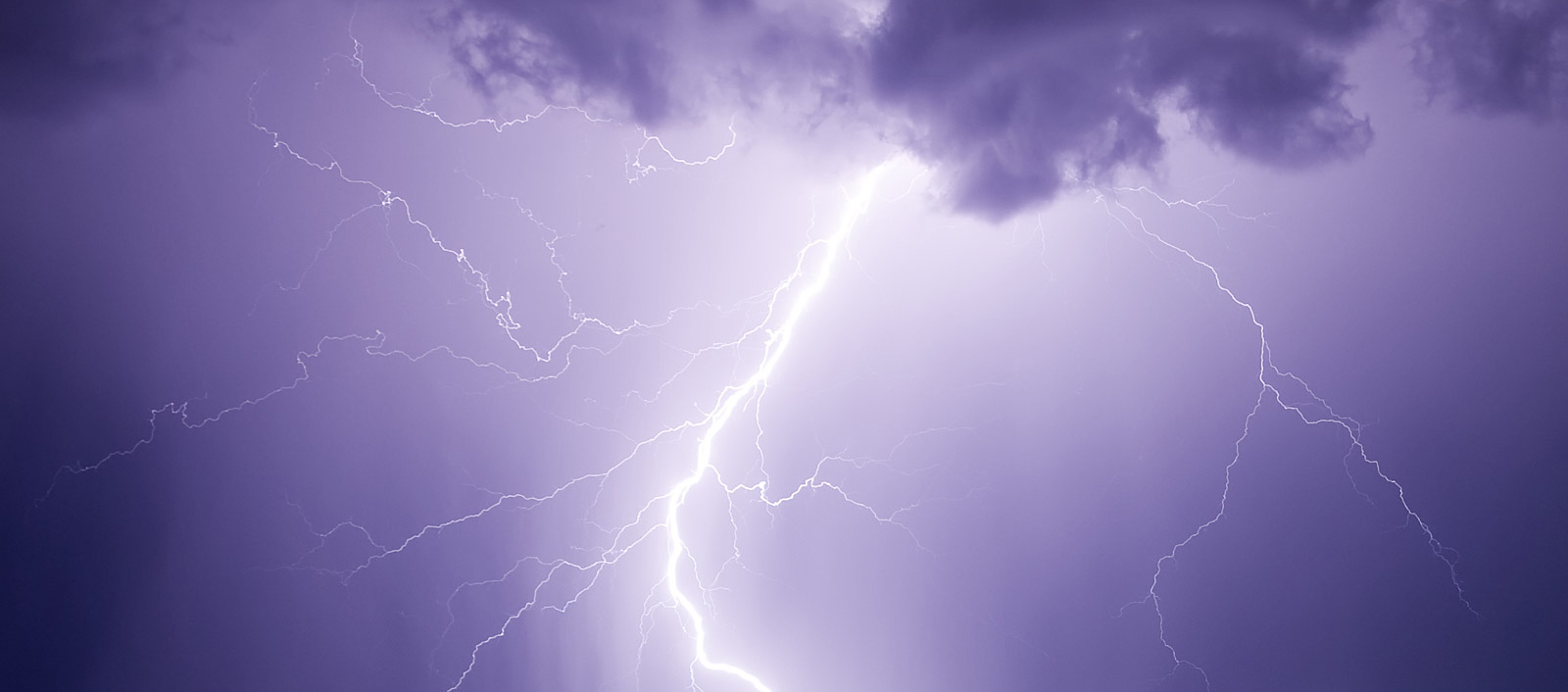
Shot Craft: Deep Focus — The Basics of Electromotive Force
To understand how electricity works, we need to break it down into its most simplistic form. This requires a journey back in time to high-school science class.

To understand how electricity works, we need to break it down into its most simplistic form. This requires a journey back in time to high-school science class, where we’ll revisit atomic theory. Don’t worry, we won’t stay there long.
Hydrogen has the simplest atomic structure of all of the elements on the periodic table. The hydrogen atom has a nucleus, with one proton at its center and one electron orbiting around it. If you’ll remember from yesteryear’s science class, the number of protons, which are positively charged, is always equal to the number of electrons, which are negatively charged, in atoms that are electrically neutral.
However, when you have one lone electron out in its own orbit — as is the case with the hydrogen atom — it can become easily dislodged. This, in turn, changes the polarity of the atom from neutral to positive. Atoms, as it turns out, don’t like to be imbalanced, so this little atom will now steal the electron from a neighboring atom to make itself neutral once again. This kicks off a chain reaction of moving electrons right on down the line, from atom to atom — and this exchange of electrons is called electromotive force.
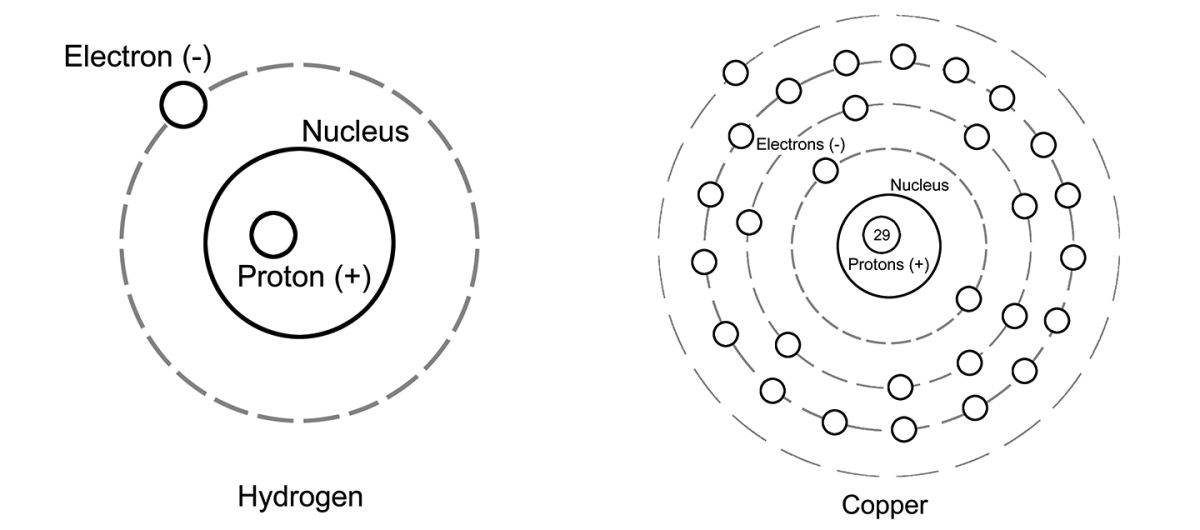
All conductors, which are materials that allow for the easy flow of electric current, have this free electron in the orbit of their individual atoms. The best conductors are metals: silver, gold, copper and aluminum (in that order, with silver being the most conductive).
Copper is one of the most common conductors used, as it offers the best balance of conductivity to cost — although aluminum was used quite heavily in the 1970s and ’80s, and in some cases can still be found. If we look at the atomic structure of copper, we’ll see that it has 29 electrons and 29 protons, but the 29th electron is in its own orbit and is therefore easily dislodged.
It’s important to remember that heat is a byproduct of electromotive force — the transfer of electrons generates heat. And in high enough amounts, of course, heat can cause fire. Mishandled, the flow of electrons through a conductor can easily cause materials around the conductor to melt or burst into flames. This makes fire one of the greatest hazards in electricity (alongside electrocution).
In a previous Shot Craft (“Keeping the Lights On,” AC March ’18), we looked at three of the principal elements of electricity: volts, amps and watts. Let’s take another look at those, along with a fourth: ohms.
If you think about the flow of electricity like the flow of water, some of these components are easier to understand. Imagine a large bucket of water with a spigot at the bottom. If the bucket is resting on the ground and you open the spigot, water will spill out onto the ground at a certain pressure. If you raise this bucket up into the air, though, and then attach a pipe to the spigot, the water pressure at the ground level is going to be greater than it was when the bucket was on the ground.
In the most simplistic terms, this is the same principle as voltage, which is the measurement of the electrical potential between two points, or the measurement of how many free electrons are available to pass from point A to point B. In other words, voltage — which, remember, is also known as electromotive force, and therefore is often abbreviated with the letter E in mathematical equations — is the measurement of the “pressure” of electrical flow.
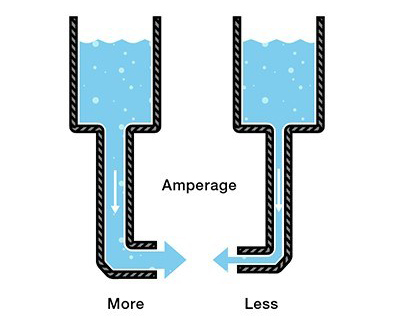
Amperage — which is also referred to as “intensity” and is often abbreviated with an I in mathematical equations — is the measurement of the capacity of flow of electrons. How many electrons are flowing past a given point at a given time? Amperage is dictated by the capacity of the conductor and is directly related to the amount of electricity ultimately produced. If you think about amperage as the diameter of the pipe connected to the bucket, it’s easier to grasp the concept. If you have a 1⁄2"-diameter pipe attached to the bucket, no matter how much pressure is behind that flow, it’s only going to allow so much water to drain at one time. If you swap out that pipe for a 1"-diameter pipe, twice the amount of water can flow through the pipe.
Wattage — aka “power,” and abbreviated with the letter P — is the measurement of the work done. Or, with our bucket analogy, it’s the amount of water you collect at ground level. That amount of water is directly proportional to the pressure and rate of flow — watts are the product of volts multiplied by amps. As an equation, that means W = V x A, or P = E x I.
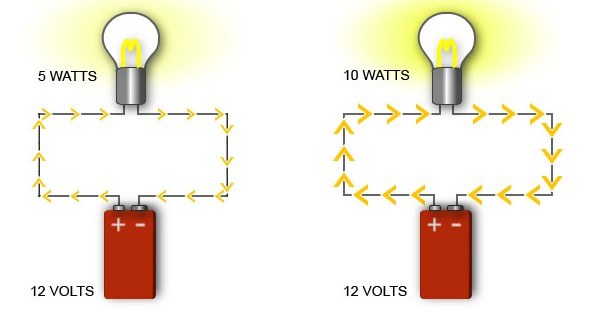
The final element is the ohm, which is often represented by the letter R in equations and is used to measure the resistance to electromotive force. All materials have some resistance to the flow of electricity. Metals (conductors) have the least resistance, whereas dry rubber, dry wood and dry air have the most resistance. Ohms can be thought of as twists and turns in the pipe coming from the bucket, reducing the flow of water, regardless of the pressure or diameter of the pipe.
One more step back into science class: Notice that I specified dry rubber, wood and air. That’s because water is two parts hydrogen and one part oxygen, and the hydrogen molecule, with its single electron, is a fantastic conductor of electricity. Now let’s walk down the hall to health class, where we’ll be reminded that the human body is 60-70 percent water — which means that people are pretty good conductors of electricity. So please, be careful when working with electricity, and avoid completing any circuits with your body!